
Solution Crystallization
We developed a spherical variant of the CuMD method to carry out nucleation simulation at constant supersaturation of the solution. Our method allows determining the crystal nucleus size and nucleation rates at constant supersaturation. As an example, we study the homogeneous nucleation of sodium chloride from its supersaturated aqueous solution.
J. Chem. Theory Comput., 2019, (ASAP) (https://doi.org/10.1021/acs.jctc.9b00795)
Research related movies YouTube link

Experiments and constant chemical potential molecular dynamics simulations are combined to investigate the solvent effects on isoniazid crystal morphology.
Chem. Engg. Sci. 2019, 31, 320-328 (https://doi.org/10.1016/j.ces.2018.10.022)
A constant chemical molecular dynamics simulation method has been developed and applied to study simultaneous growth and dissolution of urea crystal from aqueous solution.
J. Chem. Theory Comput., 2018, 14 (5), pp 2678–2683 (DOI: 10.1021/acs.jctc.8b00191)
Ligand binding/unbinding in enzymes
Atomistic molecular dynamics (MD) simulations coupled with the metadynamics technique were carried out to delineate the product (PPi.2Mg and IMP) release mechanisms from the active site of both human (Hs) and Plasmodium falciparum (Pf) hypoxanthine-guanine-(xanthine) phosphoribosyltransferase (HG(X)PRT).
J. Chem. Inf. Model. 2016, 56, 8, 1528-1538 (https://doi.org/10.1021/acs.jcim.6b00203)
Other works related to solution crystallization
Check Publications page
Atomistic molecular dynamics simulations have been carried out to delineate the mechanism of the release of product molecules phenylacetate (PAC) and carbon dioxide (CO2), from the wild-type (WT) and its G74C/C188S mutant enzymes.
J. Phys. Chem. B 2016, 120, 45, 11644-11653 (https://doi.org/10.1021/acs.jpcb.6b07034)

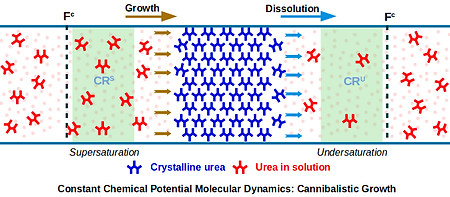
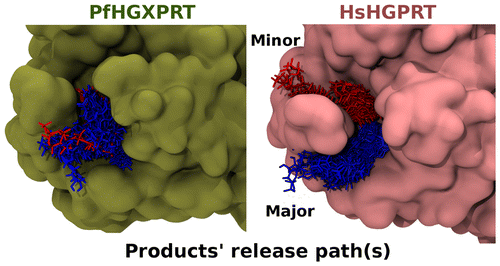

Enzyme Dynamics (in silico Mutations)
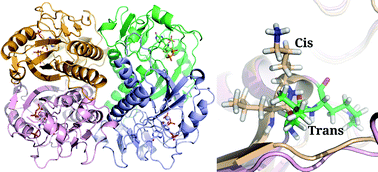
Slow ligand-induced conformational switch increases the catalytic rate in Plasmodium falciparum hypoxanthine guanine xanthine phosphoribosyltransferase. The confromational flipping from trans to cis of the Leu-Lys dipeptide has been found to be the key process in the activation of the PfHGXPRTase enzyme.
Mol. BioSyst., 2015,11, 1410-1424 (DOI: 10.1039/C5MB00136F)
Atomistic molecular dynamics simulations have been performed on two types of PfHGXPRT dimers (D1 and D3) and its tetramer in their apo and ligand-bound states. The presence of ligand molecules in subunits of the tetramer reduces the flexibility of loop X epitomizing a communication between this region and the active sites in the tetramer. Participation of this loop in the oligomerization step has been discerned based on the correlated motions observed in MD simulations.
J. Biomol. Struct. Dyn. 2016, 34, 1590-1605 (https://doi.org/10.1080/07391102.2015.1085441)
Other works related to Enzyme Dynamics
Check Publications page
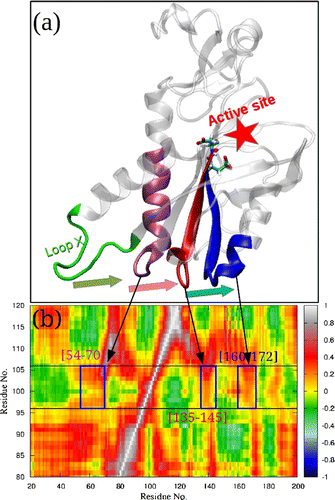

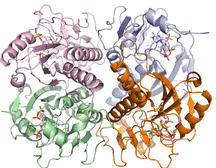
Enzymes in Ionic-liquids

Molecular dynamics simulations of TLL in aqueous solutions of a biocompatible ionic liquid (IL), cholinium glycinate (ChGly), have been carried out to investigate the microscopic reasons for the experimentally observed enhancement in the activity of TLL upon addition of a room temperature IL (RTIL), especially at an optimal concentration. The conformational switch of the R84 residues has been found to be the key step during the activation of the TLL enzyme.
J. Phys. Chem. B 2016, 120, 45, 11720-11732 (https://doi.org/10.1021/acs.jpcb.6b08534)
Gas Diffusion in Enzymes
Oxalate decarboxylase catalyzes the decarboxylation of oxalate to formate and CO2 in the presence of molecular oxygen. Herein, the transport of the product, i.e., CO2, from the reaction center to the surface of the enzyme is studied using atomistic molecular dynamics simulations. The transport of CO2 is shown to occur in a facile manner from only domain I and not from domain II, indicating that the former is likely to be the active site of the enzyme.
J. Phys. Chem. B, 2013, 117, 41, 12451-12460 (https://doi.org/10.1021/jp4074834)
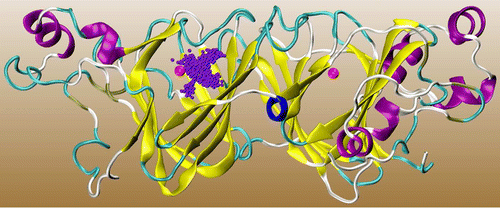
Other works related to Gas Diffusion/Protein-gas interactions
Check Publications page